Offre de thèse
LUE – Géochimie et Ecotoxicologie de deux Métaux stratégiques dans un continuum terre-mer: MODélisation des processus
Date limite de candidature
30-09-2024
Date de début de contrat
01-11-2024
Directeur de thèse
GIAMBERINI Laure
Encadrement
LIBRALATO Giovanni University of Naples Federico II (Italie), Laboratory of Hygiene: Water, Food and Environment,
Type de contrat
école doctorale
équipe
ECOSe - ECOlogie du Stresscontexte
L'objectif de neutralité carbone, fixé à 2050 par la loi énergie-climat, pour répondre à l'urgence climatique nécessite la sortie progressive des énergies fossiles et le développement des énergies renouvelables à faible émission de carbone. Ceux-ci sont plus intensifs en matériaux que les technologies traditionnelles et génèrent une augmentation de la consommation de ressources minérales telles que les métaux critiques (CM) terres rares (ETR) et le lithium (Li). Les exigences accrues pour ces MC ont déjà augmenté leur extraction, entraînant inévitablement une augmentation de leurs rejets et des pressions qui en résultent, les obligeant à être considérés comme des contaminants émergents. Malgré un nombre croissant d'études, les connaissances restent limitées sur les nombreux processus géochimiques et leurs comportements écotoxicologiques, leur spéciation, leur biodisponibilité, leur accumulation dans le biote d'eau douce et marin et leur organotropisme. La connaissance de leurs effets (éco)toxicologiques est fragmentée et pas nécessairement concluante, souvent exposome, CM et espèces dépendantesspécialité
Ecotoxicologie, Biodiversité, Ecosystèmeslaboratoire
LIEC - Laboratoire Interdisciplinaire des Environnements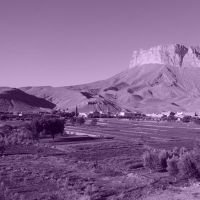
Mots clés
Gd-Li , Ecotoxicologie, Géochimie, ModélisationTKTD, Terre-Mer
Détail de l'offre
L'objectif de neutralité carbone, fixé à 2050 par la loi énergie-climat, pour répondre à l'urgence climatique nécessite la sortie progressive des énergies fossiles et le développement des énergies renouvelables à faible émission de carbone. Ceux-ci sont plus intensifs en matériaux que les technologies traditionnelles et génèrent une augmentation de la consommation de ressources minérales telles que les métaux critiques (CM) terres rares (ETR) et le lithium (Li). Les exigences accrues pour ces MC ont déjà augmenté leur extraction, entraînant inévitablement une augmentation de leurs rejets et des pressions qui en résultent, les obligeant à être considérés comme des contaminants émergents. Malgré un nombre croissant d'études, les connaissances restent limitées sur les nombreux processus géochimiques et leurs comportements écotoxicologiques, leur spéciation, leur biodisponibilité, leur accumulation dans le biote d'eau douce et marin et leur organotropisme. La connaissance de leurs effets (éco)toxicologiques est fragmentée et pas nécessairement concluante, souvent exposome, CM et espèces dépendantes. Dans un contexte environnemental d'expansion de l'utilisation des ETR et des rejets croissants, le projet propose d'évaluer leur devenir et leurs impacts environnementaux sur deux espèces emblématiques des milieux aquatiques, ultime réceptacles de contaminants. Le projet interdisciplinaire se concentrera sur le continuum terre-mer, réunissant, croisant les perspectives des chercheurs des disciplines de la géochimie environnementale, de l'écotoxicologie et de la modélisation. L'objectif est de caractériser et d'étudier les relations entre exposome et reactome au Gd et Li chez deux bivalves : un dulçaquicole (D. polymorpha) et un marin (M. galloprovincialis) et d'anticiper les risques environnementaux et éventuellement de consommation humaine dans un contexte de vide juridique concernant les seuils autorisés et les niveaux toxiques vis-à-vis des organismes vivants. Afin de valider des approches expérimentales en milieu naturel, nous étudierons la relation entre l'exposome dans des situations complexes in situ dans des sites post-minier au Canada (minéralisation Li, géochimie) et le reactome (bioaccumulation, organotropisme, fractionnement isotopique, écotoxicologie). L'étude en milieu naturel permettra de mieux comprendre l'homéostasie de ce métal dans les populations naturelles, et ainsi aider à élucider les mécanismes d'action du lithium.
Keywords
Gd-Li , Ecotoxicology, Geochemistry, TKTD modelling, land-sea continuum
Subject details
The objective of carbon neutrality, set for 2050 by the energy-climate law, in order to respond to the climate emergency requires the gradual exit of fossil fuels and the development of low-carbon renewable energies. These are more material intensive than traditional technologies and generate an increase in the consumption of mineral resources such as the so-called critical metals (CM) rare earths (ETR) and lithium (Li). The increased requirements for these MCs have already increased their extraction, inevitably leading to an increase in their releases and resulting pressures, forcing them to be considered as emerging contaminants. Despite a growing number of studies, knowledge remains limited regarding the many geochemical processes and their ecotoxicological behaviours, from their speciation to their bioavailability, their accumulation in freshwater and marine biota and their organotropism. Knowledge about their (eco)toxicological effects is fragmented and not necessarily conclusive, often exposome, CM and dependent species. In an environmental context of expansion of the use of REE and Li and increasing releases, the project proposes to evaluate their fate and environmental impacts on two emblematic species of aquatic environments, ultimate receptacles of contaminants. The interdisciplinary project will focus on the land-sea continuum, bringing together, crossing the perspectives of researchers from the disciplines of environmental geochemistry, ecotoxicology, and modelling. The objective is to characterize and study the relationships between Gd and Li exposome and reactom in two bivalves: a dulçaquicole (D. polymorpha) and a sailor (M. galloprovincialis) and to anticipate environmental and possibly human consumption risks in a context of legal vacuum concerning authorised thresholds and toxic levels vis-à-vis living organisms. In order to validate experimental approaches in the natural environment we will study the relationship between the exposome in complex in situ situations in post-mining sites in Canada (Li mineralization, geochemistry) and the reactome (bioaccumulation, organotropism, isotopic fractionation, ecotoxicology). The study in the natural environment will provide a better understanding of the homeostasis of this metal in natural populations, and thus help elucidate the mechanisms of action of lithium.
Profil du candidat
Ce projet interdisciplinaire nécessitera d'avoir un candidat ou une candidate curieux, ouvert aux différentes approches menées entre géochimie, écotoxicologie et modélisation et déjà bien formé sur les diverses méthodologies. Le candidat devra en particulier posséder de bonnes qualités techniques (connaissances de bases des concepts en écotoxicologie, en écotoxicologie expérimentale et modélisation géochimique ou écotoxicologique) et rédactionnelles. De bonnes qualités relationnelles sont également attendues pour assurer les interactions avec les différents interlocuteurs de ce projet interdisciplinaire et international.
Candidate profile
This interdisciplinary project will require a curious candidate, open to different approaches between geochemistry, ecotoxicology and modeling and already well trained on the various methodologies. In particular, the candidate must possess good technical (experimental ecotoxicology and geochemical or ecotoxicological modelling) and writing skills. Good interpersonal skills are also expected to ensure interactions with the various partners of this interdisciplinary and international project.
Référence biblio
3. Exposé scientifique du projet - Scientific project
The Energy-Climate Act adopted in 2019 sets ambitious targets for France's climate and energy policy. The law sets a target of carbon neutrality by 2050, in response to the climate emergency and the Paris Agreement, one of whose four pillars is the gradual phase-out of fossil fuels and the development of renewable energies. Low-carbon technologies are more material-intensive than traditional technologies, and energy efficiency linked to the development of renewable energies is also accompanied by increased consumption of mineral resources, including so-called critical metals (CM) or strategic metals for geological or economic reasons. Whether for electrified vehicles (cobalt, lanthanum, lithium), catalytic converters or fuel cells (platinum, palladium, rhodium), wind power (neodymium, dysprosium, terbium, etc.), civil aeronautics (neodymium, dysprosium, terbium, etc.) or energy efficiency (cobalt, lanthanum, lithium), civil aeronautics (titanium consumer) or solar photovoltaics (cadmium, indium, gallium consumer), all the innovations developed to achieve the energy transition depend on the availability of certain ores and refined metals, such as rare earths (REE) and lithium (Li).
REEs are a group of 17 elements (scandium, yttrium and 15 lanthanides), which have become essential for many cutting-edge industries in the military and medical fields, as well as for low-carbon technologies (wind turbine magnets). Their remarkable properties (high thermal stability, high electrical conductivity, strong magnetism) have enabled significant performance gains while reducing the quantity of materials consumed. Mining production of REE has almost tripled in 25 years, rising from 80,000 t in 1995 to 213,000 t in 2019. China largely dominates production (62%), followed by the United States (12%) and Burma (10%). In line with the expected increase in installed capacity, the results show an almost 2.5-fold increase in total rare earth consumption in a 4° C scenario, and a more than 10-fold increase in a 2° C scenario by 2050 (Hache et al 2021b, IFPEN 2020).
As for lithium, in addition to being very light, it has a very low electronegativity, making it the material of choice for batteries that enable reversible energy storage. With the development of Li-ion technologies, it has found a strong outlet in this sector, whether for portable electronics (notably cell phones and laptops) or, more recently, for electric vehicles. Today, two regions - Australia and Latin America - account for 80% of mining production. China controls between 50% and 89% of the world's refined Li production, and accounts for 61% of global Li-ion battery production capacity. Global Li production has increased 3-fold in the space of a decade, from 25,000 t in 2010 to over 82,000 t in 2020. In recent years, demand for Li has grown at a steady rate of around 20% a year. This trend looks set to continue with the rise of electric mobility, which is in demand as part of the ecological transition, accounting for just 45% of Li demand in 2021 and is expected to account for up to 90% of demand by 2030. Demand for Li is set to increase by a factor of 5 to 7 over the next decade, with demand estimated at over 680 kt in a zero-emission scenario. There is no shortage of information on the location, uses and concentrations of Li in soils, minerals and clays, but the literature is less abundant concerning knowledge of Li levels in surface waters (Hache et al 2018; 2021a).
The growing demand for these two MC has already led to an increase in their extraction, inevitably leading to an accentuation of their discharge and the resulting pressures, which obliges/allows these metals to be considered as emerging contaminants. Despite industrial practices and processes that seek to be more respectful of the environment, and the promotion of more responsible development of mineral resources (Plante et al., 2023), the environmental footprint of mining is not negligible. Every stage in their life cycle, from extraction to recycling, transport and use, leads to enrichment in all environmental compartments, the ultimate receptacle of which is the aquatic ecosystem, first freshwater and then marine. These ecosystems are affected not only in urban areas, but also in more natural areas close to mining zones (Roy et al 2022; Gao et al 2023).
The anthropogenic impact on the transport of colloidal or dissolved REEs in rivers is now well established. In recent years, enrichments of many REEs have been observed worldwide particularly in Gd, Ce, Sm, Eu, Tm and Yb (Hissler et al 2022; Gao et al 2023). In their recent review, (Ebrahimi & Barbieri 2019) show that gadolinium (Gd) anomaly is now ubiquitous and has been increasing for 30 years in many European countries including France and Italy, but also in the USA and Australia. Gd is found in surface water, groundwater and tap water. In San Francisco Bay, its anomalies of 10 pmol/kg in 1993 doubled in 2000, reaching 100 pmol/kg in 2013. The level of anthropogenic Gd (Gdanth) in the Moselle and its tributaries is close to or above the value of 20 ng/L reported in the literature to cause adverse cellular effects (Louis et al, 2020; Parant et al 2018). This portion of anthropogenic metal, detected in all aquatic systems, comes from Gd used in the form of Contrast Agent (Gd-AC) in Magnetic Resonance Imaging examinations, which is virtually untreated in wastewater treatment plants after excretion by patients and is discharged in its entirety into the environment in a context of legal vacuum concerning authorized thresholds in REE and toxic levels with respect to living organisms.
In general, REE concentrations measured in freshwater decrease on reaching brackish waters. Different processes take place on the land-sea continuum, leading to changes in REE abundance and/or speciation. The mixing of fresh and salt water in estuarine zones leads to precipitation of lanthanides, followed by subsequent sedimentation with removal rates ranging from 30% to 90%. In coastal waters, particularly complex transition zones, the highest concentrations of REE are found at the surface, decreasing with depth and open sea (Neira et al 2022). While studies on the environmental impact and ecotoxicology of REEs have progressed over the last decade, few studies have been published on marine species (17 references between 2003 and 2020, Piarulli et al, 2021), and many barriers remain to the response of all the elements in this family. We know little about many geochemical processes and their ecotoxicological behavior, from speciation to bioavailability, accumulation in freshwater and marine biota and organotropism. Knowledge of their (eco)toxicological effects is fragmented and not necessarily conclusive, often exposome, REE and species dependent (Gonzalez et al 2014; Moreira et al 2020; Arienzo et al 2022; Neira et al 2022, Lachaux et al 2022a,b; 2023; Revel et al 2023; Pagano et al 2023). Effects such as abnormal development, cellular and tissue damage, oxidative stress and neurotoxicity have been reported in living organisms. Currently, the environmental risk is proven but limited to hot spot areas of contamination such as industrial, hospital or sewage plant effluent discharge zones and mining activity sites (Gonzalez et al 2015; Lachaux et al 2022b).
The main knowledge gaps relate to 1) harmonization of data between the disciplines of geochemistry and biology 2) behavior and fate of REEs 3) toxicological effects on organisms 4) ecological impact and risk assessment of different REEs (Piarulli et al, 2021). Concerning (Gdanth) studied in this project in CA form, its potential toxicity may represent a problem linked to the size proximity of its ionic radius to that of Ca inducing calcium channel blockade. Moreover, with its higher affinity, it competes with Ca2+ in physiological processes, leading to reduced neuromuscular transmission and interference in enzymatic and membrane processes. Although some studies have shown the ability of Gd-AC to accumulate in certain aquatic organisms (Perrat et al 2017), the overall processes of bioavailability, bioaccumulation in the biota, induced effects and risks to humans are still very poorly investigated.
With regard to Li in coastal systems, Thibon et al (2021a) and Barbosa et al (2023) report on 60 publications since the 1960s. Of these, only 37 report data on Li concentrations, which are difficult to compare and require large-scale monitoring. Marine Li levels are fairly homogeneous, around 180 µg/L, with the highest levels found in the Atlantic Ocean (220 µg/L). Along food webs, Li tends to show biodilution, with the highest concentrations measured in bivalves, which may represent a risk for humans (Thibon et al 2021a; Barbosa et al 2023a,b), especially as Li is not currently taken into account in marine biomonitoring programs (Thibon et al 2021b). A total of 84 publications are reported in freshwater environments, and the rare occurrence data in surface waters show low levels, generally between 1 and 10 μg/L, but as high as 170 μg/L without knowing how levels have recently evolved. Streams in Li-rich regions in northern Chile can contain up to 5.2 mg/L, and groundwater up to 500 mg/L (Aral and Vecchio, 2011). Furthermore, spa sites advertising their Li-rich waters across the globe report levels ranging from 1 to 10 mg/L.
In existing literature, the environmental impact of Li is only partially documented. Li from waste disposal sites in the USA contaminates groundwater at levels toxic to some freshwater organisms (50 to 170 μg/L), and well above those in most rivers in the USA and worldwide (~1.5 μg/L). The biological effects studied on this element mainly concern therapeutic actions and developmental biology. Indeed, in the healthcare field, Li remains the reference treatment for bipolar disorders. Numerous studies are therefore available on its mechanisms of action on mammals in the context of therapeutic effect, even if this subject is still the subject of debate, given the divergent effects between studies.
Most of Li's ecotoxicity data concern acute toxicity in fish. Few chronic toxicity data are available, although they are more representative of environmental conditions. Most studies have been carried out with Li sulfate or chloride, and little information is available on the toxicity of other salts used in industry, such as carbonate or hydroxide. Toxicological data are highly variable, showing effective concentrations at 50% (EC50) in acute toxicity at least 10 times higher than average environmental concentrations (for review, Barbosa et al 2023a, Aral and Vecchio 2011, Siebel et al 2014). The maximum concentrations currently measured in the various ecosystems are then closer to the available chronic EC50s, and the PNEC (Predictive No Effect Concentration) values established in standardized freshwater are between 2 and 10 mg/L depending on the Li salt. Li toxicity may be salt-dependent and masked by the presence of sodium. These two factors are rarely taken into account, making interpretation and intercomparison of results difficult and raising questions about individual toxicity. Available studies on aquatic organisms show inhibition of growth, oxidative stress, developmental toxicity, teratogenesis and neurotoxicity.
In an environmental context of expansion of the use of REE and lithium and increasing releases, the project proposes to evaluate their fate and environmental impacts on two species of invertebrates emblematic of aquatic environments. This interdisciplinary project will focus on the land-sea continuum, bringing together researchers from the fields of environmental geochemistry, ecotoxicology, molecular biology and modeling. The aim is to characterize and study the relationships between the exposome, metallome, metabolome and reactome in two bivalves: one freshwater (Dreissena polymorpha) and one marine (Mytilus galloprovincialis), and to anticipate environmental risks. This type of project, which is highly integrated in disciplinary and spatial terms, does not exist or exists only to a limited extent for other, more conventional metals, and is therefore completely innovative for REE and Li, for which no environmental standards have yet been established. The main challenge is to describe, characterize and formalize the links between exposure to contaminants (physico-chemical characterization of environments, geochemistry, speciation, bioavailability, bioaccumulation, organotropism, cellular distribution) and the biological responses observed in organisms. Laboratory experiments will provide answers to the following questions:
1. What is the fate and bioavailability of Gd-AC and Li in fresh and marine waters?
2. What is their transfer, bioaccumulation and distribution in biota?
3. What are the molecular and cellular mechanisms and effects induced in exposed organisms?
4. How can we predict the exposure of aquatic organisms to Gd-AC and Li, and the transfer levels at risk for bivalves, by developing mathematical and statistical approaches?
Field experiments will provide answers to the following questions :
1. What is the potential risk associated with the transfer of Li from various mineralizations to biota and its potential biomagnification
2. What is the anthropogenic impact of Li in areas of exploitation and industrial use
The project will comprise 5 interconnected tasks involving the highly complementary partners from France, Canada and Italy. The combination of the different approaches developed will provide a systemic view of the interaction between Gd-AC and Li - aquatic organisms, and will lead to a better understanding of their ecotoxicity, toxicokinetics and toxicodynamics, improved environmental risk assessments, predictive modeling and the development and implementation of more targeted management measures.
Task 1: Characterization of the exposome of the two bivalve species (partners 1, 2 & 3)
The fate, speciation and bioavailability of the 2 CM will be studied and modeled. The exposure will be made more complex for greater environmental realism by adding organic matter known to influence metal speciation and bioavailability.
Since the late 1970s, the zebra mussel D. polymorpha has been used as the inland counterpart of Mytilus spp. in biomonitoring programs, due to their similar ecological characteristics and close phylogenetic affinity. Filter-feeding, sedentary bivalves are well-known key bioindicator species in aquatic environments, due to their ability to accumulate pollutants in their soft tissues and to demonstrate induced effects. The exposure experiments will take place in Lab partner 1 for the freshwater species and Lab partner 2 for the marine species. Each species will be exposed for one week to 2 concentrations of each metal representing low and high environmental contamination, taking into account available toxicity data. This will be followed by a depuration phase in a metal-free environment for 2 weeks. Because of their important uses, particularly in the medical field, and the environmental issues involved, the metals selected for this project are Gd-AC and Li in its carbonate form, which is the mineralogical form mainly produced and the second most toxic (LIEC personal data). Samples of organisms and media will be taken at 5 time steps during the exposure phase and 6 during the depuration phase. Concentrations of Gd & Li as well as those of major elements with which they may be associated (Na, Mg, Si, Al, P, Ca, Mn, Fe) will be monitored in the media and the various tissue and cell compartments using ICP-MS. Their speciations will be assessed using thermodynamic equilibrium models (e.g. PHREEQC-Model VII, Visual MINTEQ). To strengthen the link with biological effects and compare geochemical and toxicological approaches, metal pollution indexes can be calculated from geochemical environmental data, a speciality of partner 3 (Edahbi et al 2018).
Task 2 : Bioaccumulation, organotropism and cellular distribution of the metals Gd and Li (part. 1 & 4).
The study of bioaccumulation enables us to understand the link between exposure concentration and the toxic effects engendered. Bioaccumulation involves various processes grouped together under the term ADME (Absorption, Distribution, Metabolization, Elimination) which depend on the physico-chemical properties of the contaminants, the species under consideration and environmental conditions. Toxic-kinetic (TK) models are used to formalize and describe these processes, whether at the scale of the whole organism (single-compartment TK models) or at the finer scale of organs/tissues (multi-compartment TK models). Today, the study of ADME processes in aquatic organisms is mainly carried out on whole organisms. However, recent innovative work on gammarids (Gestin et al 2021; 2023) (partner 4) has demonstrated the added value of exploring a finer scale, at the level of different organs/tissues that have specific roles in ADME processes. The same methodology will be used here on the 2 bivalve species, based on bioaccumulation data measured in gills, digestive glands and other soft tissues (Task 1). In the first instance, single-compartment TK models will be fitted to the concentrations bioaccumulated by each organ/tissue independently, so as to compare the accumulation and depuration capacities of each organ independently of the others. Secondly, in order to describe the organotropism and internal fate of TMEs, a multi-compartment TK model will be developed (partner 4) in both bivalve species and fitted, by Bayesian inference, to bioaccumulation data measured in all organs/tissues simultaneously. The iterative model selection procedure developed by partner 4 (Gestin et al., 2023) will ultimately enable us to describe the fate of Gd and Li in bivalves and propose a hypothesis on the general management of each metal over time.
In order to reinforce the results obtained on the metabolism of 2 MC in model species, sub-cellular fractionation measurements of trace metals will be undertaken, enabling the separation of detoxified forms and fractions contributing to toxic effects. Such studies may also reveal the trace metal management strategies employed by aquatic organisms. In addition, detailed characterizations of biomolecules involved in detoxification or metal-sensitive responses are needed to better understand their intracellular speciation. A sub-cellular partitioning protocol including differential centrifugation, NaOH digestion and heat treatment steps will be applied to determine the concentrations of Gd and Li in the 'detoxified metals' compartment from the so-called 'sensitive' ones such as in heat-sensitive protein fractions, mitochondria and other organelles (Wallace et al. 2003). The sub-cellular fraction will then be integrated into the multi-compartment TK model developed for each species. This first approach to modelling the multi-compartment (organism, tissue, cellular fractions) bioaccumulation of Gd and Li in two bivalve species (freshwater and marine) may provide innovative information for the diagnosis of the chemical quality of water bodies required by European regulations, by proposing improved methods for estimating bioaccumulation metrics.
Task 3: Determination of the toxic mode of action of Gd and Li and integrated assessment of biological effects at molecular, cellular and individual levels (Partners 1, 2)
The metabolic profile of an individual, being the result of all metabolic activities, provides a global vision of metabolic pathways and physiological functions impacted by exposure to a contaminant. This can therefore provide information on the mechanism of action of the contaminant and on the physiological states of the resulting organisms. An integrative metabolomic approach (concentration and target exposure time) will be conducted by combining two complementary non-target methods (1) Nuclear Magnetic Resonance (NMR) and (2) High Resolution Mass Spectrometry (HRMS), both allowing to detect changes in the abundance of products of the primary metabolism. This innovative combination increases the possibility of revealing disturbed metabolites (improved detection and annotation of metabolites) thus allowing a broader questioning of the fundamental biochemical pathways involved in the response to exposure to MC (Bhinderwala et al 2018; Prud'homme et al. 2020). At the cellular level, a battery of about 25 biomarkers related to major physiological functions (antitoxic and antioxidant defenses, immune system, energy metabolism, hormonal system, osmoregulation, ionoregulation, reproduction) will be monitored in parallel with exposure parameters (concentrations in medium and tissue). Finally, at the individual level the filtration activity will be evaluated in both bivalves and will provide information on the ecosystem function of the organisms chosen in their respective environments.
Task 4: Lithium-Health-Environment: Establish the basic geochemical and environmental conditions and trophic transfer of lithium in freshwater ecosystems.
The aim is to validate experimental approaches in the natural environment by studying the relationship between the exposome in complexe in situ situations (Li mineralization, geochemistry) and the reactome (bioaccumulation, organotropism, isotopic fractionation, ecotoxicology). The study in the natural environment will provide a better understanding of the homeostasis of this metal in natural populations, and thus help elucidate the mechanisms of action of lithium.
To date, the assessment of lithium concentrations in freshwater organisms and their controlling factors have not been investigated, and their possible evolution along food webs remains unknown or poorly documented (Thibon et al 2021b). Li homeostasis has yet to be fully elucidated in animal organisms living at different trophic levels. Variations in Li isotope fractionation in organisms with different diets or life-history traits could be an explanatory element. Indeed, some studies show that the 6Li isotope is more biologically reactive than 7Li due to its 15% mass difference, and therefore has a higher diffusion.
In Canada, partner 3, Benoit Plante, IRME - UQAT, is conducting research on a former Québec Lithium Corporation mine site at La Corne (Québec, Canada), operated between 1955 and 1965, whose tailings contain high levels of lithium that may now be of interest again. The geochemistry of these spodumene-pegmatite tailings is completely different from that of Li-rich deposits in France (studied in a previous project).
At this site, geochemically characterized by IRME, samples of water, sediment and organisms representative of freshwater trophic chains will be collected and analyzed for Li content. Animal species will be selected according to trophic level, taxonomic group and habitat. Where possible, and depending on the size of the organisms, we will monitor Li content in different organs to track metal organotropism (muscle, liver, gills), and study natural variations in 7Li/6Li ratios and fractionation in animal organisms. Understanding the distribution of Li in the various abiotic and biotic compartments of a post-mining situation will provide useful information for setting up more respectful mining processes for (pre-)mining sites and/or remediation actions.
Task 5: Remediation trajectories: Cross-reflection and synthesis (All partners)
The 4 partners have a very good knowledge of the entire life cycle of MCs from mining to recycling and belong to networks comprising the different scientific and socio-economic stakeholders in the fields. They will be able to cross their perspectives in an interdisciplinary approach and synthesize new approaches concerning innovative solutions of use, abatement and, more broadly, reduction of mineral resource pressures on aquatic ecosystems from the source. Indeed, in the context of energy and ecological transitions, we must first promote a more responsible use of mineral resources. In this context and with an interdisciplinary and integrated vision, they may also be able to make recommendations to the various stakeholders.
The consortium formed by the 4 partners has all the skills to carry out interdisciplinary and innovative research in the field of environmental sciences, on the whole life cycle of the two strategic metals and on two abiotic and biotic continuums, first Terre-Mer for the spatial approach and then Exposome-Réactome (via metalloma and metabolioma) for the ecotoxicological approach. Partners 2, 3 and 4 who do not know each other are part of the LIEC networks but no research project has been formalized between them and this project will be an opportunity to formalize new collaborations. The UMR LBBE will bring the whole dimension of mathematical formalism of modeling to the ecotoxicological approaches developed by the LIEC.
Partner 1: UMR 7360 LIEC. The primary objective of LIEC is to understand the functioning of continental ecosystems that are highly disturbed by human activity, with the aim of their rehabilitation. For this purpose, it implements interdisciplinary research combining concepts and methods of environmental mineralogy, soil science, microbial ecology, colloidal physico-chemistry, ecotoxicology, functional ecology. The Ecology of Stress team in which L. Giamberini is specialized in (1) risk assessment & biomonitoring whose challenges are to improve current approaches and develop new methodologies and (2) the study of the propagation of observed effects at low levels of biological organization that may have temporal and spatial impacts on populations and ecosystems; and (3) the study of mechanisms of impairment of the functioning of ecosystems subject to multiple stresses.
Partner 2: University of Naples Federico II. G. Libralato's main research interests are related to applied environmental hygiene, including aquatic (eco)toxicology, nano (eco)toxicology, toxic effects on populations, factors modifying toxicity, inorganic water chemistry, wastewater treatment, soil and sediment remediation, by-product recovery and reuse, and water reuse
Partner 3 : UQAT is located in the heart of a world-class mining centre and is the only university in Quebec that has made the mining sector a distinctive element of its contribution to science and technology more than 30 years ago. With its Institute for Research in Mining and the Environment (IRME), the University can count on an internationally recognized « mining and environment » pole of fame. Benoit Plante, Institutional Research Chair in Environmental Geochemistry of Critical and Strategic Mineral Resources, is developing his research in the fields of environmental geochemistry in a mining context, prediction of geochemical behaviour of contaminated mine tailings, acid and neutral mine drainages.
Partner 4: Université Claude Bernard Lyon I, UMR LBBE. At LBBE, Christelle Lopes conducts her research in the MEPS team (Predictive Modeling and Ecotoxicology) whose activities aim to propose integrated mathematical and statistical modeling approaches to predict the effects of environmental disturbances at different levels of biological organization, as well as their consequences (functional and evolutionary) individual fitness, population, community and/or ecosystem dynamics. In order to obtain generic models with strong predictive power, the team focuses in particular on understanding the operation and not only on the extensive description of the complex systems considered. His research has contributed to the development of the MOSAIC platform (MOdeling and StAtistical tools for ecotoxICology, http://mosaic.univ-lyon1.fr) which offers a collection of data analysis and modelling tools in ecotoxicology.
Cited References (Consortium references in blue)
Aral H, Vecchio-Sadus A. 2011. Lithium: environmental pollution and health effects. Encyclopedia of Environmental Health, pp. 116–125
Arienzo M, Ferrara L, Trifuoggi M, Toscanesi M. 2022. Advances in the Fate of Rare Earth Elements, REE In Transitional Environments: Coasts and Estuaries. Water, 14, 401.
Barbosa H, Soares AMVM, Pereira E, Freitas R, 2023a. Lithium: a review on concentrations and impacts in marine and coastal systems. Sci. Total Environ., 159374
Barbosa H, Soares AMVM, Pereira E, Freitas R, 2023b. Are the consequences of lithium in marine clams enhanced by climate change? Environ. Pollut., 121416
Bhinderwala F, Wase N, DiRusso C, Powers R. 2018. Combining mass spectrometry and NMR improves metabolite detection and annotation. J Proteome Res 17(11):4017-4022.
Ebrahimi P & Barbieri M. 2019. Gadolinium as an Emerging Microcontaminant in Water Resources: Threats and Opportunities Geosciences, 9, 93
Edahbi M, Plante B, Benzaazoua M, Pelletier M. 2018. Geochemistry of rare earth elements within waste rocks from the Montviel carbonatite deposit, Quebec, Canada Environmental Sciences and Pollution Research. 25, 11, pp.10997-11010
Gao X, Han G, Liu J, Zhang S. 2023. Spatial Distribution and Sources of Rare Earth Elements in Urban River Water: The Indicators of Anthropogenic Inputs. Water, 15, 654
Gestin O, Lacoue-Labarthe T, Coquery M, Delorme N, Garnero,L, Dherret L, Ciccia T, Geffard O & Lopes C. 2021. One andmulti-compartments toxico-kinetic modeling to understand metals' organotropism and fate in Gammarus fossarum. Environment International, 156.
Gestin O, Lacoue-Labarthe T, Delorme N, Garnero L, Geffard O et Lopes C. 2023. Influence of the exposure concentration of dissolved cadmium on its organotropism, toxicokinetic and fate in Gammarus fossarum. Environment International, 171.
Gonzalez V, Vignati DAL, Leyval C, Giamberini L, 2014. Environmental fate and ecotoxicity of lanthanides: are they a uniform group beyond chemistry? Environ. Int. 71, 148-157.
Hache E, Barnet C, Seck GS 2021a. Le lithium dans la transition énergétique : au-delà de la question des ressources ? », Les métaux dans la transition énergétique, n° 4, IFPEN, Février 2021.
Hache E, Barnet Seck GS 2021b. Les terres rares dans la transition énergétique : quelles menaces sur les « vitamines de l'ère moderne ? Les métaux dans la transition énergétique, n° 3, IFPEN, Janvier 2021.
Hache E, Bonnet C, Seck GS & Simoen M. 2018. Les matériaux de la transition énergétique : le lithium. The Conversation
Hissler C, Montarges-Pelletier E, Kanbar HJ, Le Meur M and Gauthier C. 2023. Impact of past steel-making activities on lanthanides and Y (REY) fractionation and potential mobility in riverbank sediments. Front. Earth Sci. 10:1056919
IFP Energies nouvelles (IFPEN). 2020. Les métaux dans la transition énergétique. Publication des conclusions du projet GENERATE 18.06.2020 The Conversation
Lachaux N, Catrouillet C, Marsac R, Poirier L, Pain-Devin S, Gross E M,et al. 2022. Implications of speciation on rare earth element toxicity: A focus on organic matter influence in Daphnia magna standard test. Environ. Pollut. 307,119554.
Lachaux N, Cossu-Leguille C, Poirier L, Gross EM, Giamberini L. 2022b. Integrated environmental risk assessment of rare earth elements mixture on aquatic ecosystems. Front. Environ. Sci., Sec. Toxicology, Pollution and the Environment, Volume 10
Lachaux L, Otero-Farina A, Minguez L, Sohm B, Retif J, Chatel A, Poirier L, Devin S, Pain-Devin S, Gross EM, Giamberini L. 2023. Fate, subcellular distribution and biological effects of rare earth elements in a freshwater bivalve under complex exposure. Science of the Total Environment 905
Louis P. et al. 2020 Understanding Rare Earth Elements concentrations, anomalies and fluxes at the river basin scale: The Moselle River (France) as a case study. Science of the Total Environment 742 140619
Moreira A, Henriques B, Leite C, Libralato G, Pereir, E, Freitas R.2020. Potential impacts of lanthanum and yttrium through embryotoxicity assays with Crassostrea gigas. ECOLOGICAL INDICATORS. 108: 105687. [10.1016/j.ecolind.2019.105687]
Neira P, Romero-Freire A, Basallote MD, Qiu H, Cobelo-García A, Canovas CR, 2022. Review of the concentration, bioaccumulation, and effects of lanthanides in marine systems. Front. Mar. Sci. 9.
Pagano G, PrimoB, Apostolos A, Libralato G, Guida M, Trifuoggi M.2023. Hormetic Effects of Cerium, Lanthanum and Their Combination at Sub-micromolar Concentrations in Sea Urchin Sperm. BULLETIN OF ENVIRONMENTAL CONTAMINATION AND TOXICOLOGY 110:3(2023), p. 65.
Parant M, Perrat E, Wagner P, Rosin C, Py JS, Cossu-Leguille C. 2018. Variations of anthropogenic gadolinium in rivers close to wastewater treatment plant discharges. Environ. Sci. Pollut. Res.
Perrat E, Parant M, Py JS, Rosin C, Cossu-Leguille C, 2017. Bioaccumulation of gadolinium in freshwater bivalves. Environ. Sci. Pollut. Res. 24, 12405–12415.
Piarulli S, Hansen BH, Ciesielski T, Zocher AL, Malzahn A, Olsvik PA, Sonne C, Nordtug T, Munro Jenssen BM, Booth AM, Farkas J 2021. Sources, distribution and effects of rare earth elements in the marine environment: Current knowledge and research gaps. Environmental Pollution 291 118230
Plante B, Pabst T, Wilson D. 2023. Editorial for Special Issue “Environmental Geochemistry in the Mining Environment”. Minerals, 13, 112.
Prud'homme SM, Hani YMI, Cox N, et al, 2020. The zebra mussel (Dreissena polymorpha) as a model organism for ecotoxicological studies: A prior 1H NMR spectrum interpretation of a whole body extract for metabolism monitoring. Metabolites 10(6):256.
Revel M, Medjoubi K, Rivard C, Vantelon D, Hursthouse A, Heise S. 2023. Determination of the distribution of rare earth elements La and Gd in Daphnia magna via micro and nano-SXRF imaging Environ. Sci.: Processes Impacts, 25, 1288–1297
Roy T, Plante B, Benzaazoua M, Demers I, Coudert L, Turcotte S. 2022. Geochemistry of decades-old spodumene mine tailings under a humid continental climate. Applied Geochemistry 146 (2022) 105481
Siebel AM, Vianna MR, Carla DB. 2014. Pharmacological and Toxicological Effects of Lithium in Zebrafish. ACS Chem. Neurosci. 2014, 5, 468−476
Thibon F, L. Weppe, N. Vigier , C. Churlaud , T. Lacoue-Labarthe , M. Metian , Y. Cherel, P. Bustamante 2021a. Large-scale survey of lithium concentrations in marine organisms. F. Science of the Total Environment 751
Thibon F, Metian M, Oberhänsli F, Montanes M, Vassileva E, et al. 2021b. Bioaccumulation of Lithium Isotopes in Mussel Soft Tissues and Implications for Coastal Environments. ACS Earth and Space Chemistry, ACS, 5(6), pp. 1407-1417
Wallace W, Lee B, Luoma S, 2003. Subcellular compartmentalization of Cd and Zn in two bivalves. I. Significance of metal-sensitive fractions (MSF) and biologically detoxified metal (BDM). Mar. Ecol. Prog. Ser. 249, 183–197.